DEVELOPMENT OF CHLOROPLAST MICROSATELLITE MARKERS FOR Bruguiera hainesii C.G.ROGERS (RHIZOPHORACEAE) IN THE CON DAO NATIONAL PARK, BA RIA - VUNG TAU PROVINCE, VIETNAM
Joint Vietnam-Russia Tropical Science and Technology Research Center
63 Nguyen Van Huyen, Nghia Do, Cau Giay, Hanoi, Vietnam
Main Article Content
Abstract
Mangroves consist of various tropical trees or woody shrubs like plants growing at the interface between sea and land zones and form an ecologically important ecosystem. Bruguiera hainesii C. G. Rogers belonging to the family (Rhizophoraceae) is a true mangrove tree. This species was discovered in the Dam Quoc area, Hon Ba islands, Con Dao National Park, Ba Ria-Vung Tau Province. However, studies on the genetic diversity of B. hainesii are limited. Hence, this study was initiated to develop chloroplast microsatellite (cpSSR) markers and applied them to evaluate the genetic diversity of B. hainesii. A total of 133 cpSSRs markers were identified from the chloroplast genome of B. hainesii. Among all cpSSR loci, mononucleotide markers were the most abundant (91%), followed by dinucleotide (8.3%), and trinucleotide (0.7%). In total, 9 newly developed cpSSR markers with polymorphism and good stability were selected for genetic diversity analyses of 7 B. hainesii samples. These cpSSRs amplified a total of 21 alleles. We report low levels of genetic diversity of B. hainesii with Na = 2.3, Ne = 1.42, I = 0.42, Ho = 0.25, and He = 0.27. Our study not only provided a batch of efficient genetic markers for research on B. hainesii but also laid an important foundation for the breeding and conservation of B. hainesii.
Keywords
Bruguiera hainesii C. G. Rogers, mangrove species, chloroplast microsatellite markers (cpSSR), genetics diversity, đa dạng di truyền, loài ngập mặn, microsatellite lục lạp, Vẹt hainesii
Article Details
1. INTRODUCTION
Mangrove plays important roles in coastal protection, erosion control, raw material, herbal, tourist, and study places [1]. Still, in recent decades, mangroves in Vietnam have decreased for many reasons mainly is economic development purposes [2]. The Con Dao mangrove ecosystem on the China sea coastline of Vietnam is a unique place that has primary forest remaining in the country. There are 45 mangrove species in Con Dao National Park, of which 35 tree species, shrub, and climber are 5 species for each. There are 26 true mangrove species belonging to 11 families; and 19 associated mangrove species in 15 families [2]. Bruguiera hainesii C.G.Rogers, is a true mangrove species classified as ‘‘Critically Endangered (CR)’’ within the IUCN Red List of threatened species [3]. It is a species discovered in the Dam Quoc area, Hon Ba islands, Con Dao National Park with very small populations (only 7 individuals). The species is a tree that can grow to a height of 15 metres. The flowers are observed from January to March and are concentrated in clusters with 2-3 flowers on stalks [4].
Information regarding the ecological and genetic diversity of populations is necessary for the conservation and management of a species [5]. Powerful biological techniques are necessary to acquire such information, particularly a more comprehensive understanding of genetic processes. The profuse expression of phenotypes and genotypes in plants is known as genetic diversity. The polymorphic genes lead to the presence of heterozygosity genotypes in the population. When confronted with environmental changes, populations are capable of adapting to other populations due to the diverse genotypes of their members. Heterosis is established through genetic diversity.
Molecular marker technology has been recognised as a highly effective method of genetic analysis for some mangrove species [6-8]. It has also substantially facilitated the evaluation of the genetic resources of plants and the level of genetic diversity in tree species [6]. Chloroplast simple sequence repeats (cpSSRs) are a molecular marker technology that has been developed in recent years and is relatively new and effective [9]. Due to their codominant nature, high polymorphism, uniparental inheritance through chloroplast DNA (cpDNA), and absence of sexual recombination, cpSSRs are the optimal markers for population genetic diversity evaluation, population structure analysis, and phylogenetics. To date, the genetic diversity and population structure in B. hainesii have been largely unexamined. Previous research has detected the sequences chloroplast genome of B. hainesii and phylogenetic analysis with associated species [10-13]. Numerous published studies have attempted to identify the genetic diversity of some mangrove species using molecular markers, including RAPD (Random Amplified Polymorphic DNA) for genetic differentiation between B. gymnorhiza and B. sexangula in Sri Lanka [7], microsatellite (SSRs) for genetic diversity and structure of B. gymnorrhiza and Kandelia obovate [6, 8], and chloroplast microsatellite (cpSSR) markers for B. gymnorrhiza, Kandelia candel, and Rhizophora stylosa, as well as other mangrove species [14]. It is surprising that no research has surveyed the genetic diversity and population structure of B. hainesii using cpSSR markers. The protection and utilisation of the valuable genetic resources concealed in B. hainesii have been impeded by this dearth of research.
In the current study, we identify and develop novel chloroplast microsatellite markers (cpSSR) in B. hainesii and assess its level of genetic diversity. Microsatellite markers have been widely used for genetic diversity analyses because of their co-dominant inheritance and high degree of polymorphism. Following this, the study's findings are incorporated into this species' management decisions, conservation efforts and restoration efforts.
2. MATERIALS AND METHODS
2.1. Plant Material
Sampling was carried out along the coasts of Hon Ba islands, Con Dao National Park, where B. hainesii grow abundantly in saltwater regions. We collected seven samples (VH1 to VH7) from natural populations of B. hainesii in 2023 and 2024 (Fig. 1). Leaf samples were collected randomly from individual trees, cleaned, and then transferred to the Laboratory of the Joint Vietnam-Russia Tropical Science and Technology Research Center, stored at -30°C for DNA extraction.
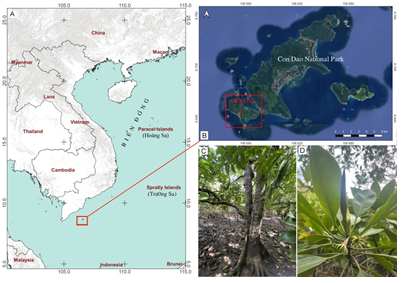
Figure 1. Map of field survey locations and geographic distributions of B. hainesii in the study.
Map showing the collection sites (A, B); adult plant (C); leaves, and flower (D)
2.2. Chloroplast microsatellite marker development
We searched the complete chloroplast genome of B. hainesii [11] (GenBank: OR086085) for microsatellite loci. cpSSR markers were identified using MISA software [15]. We applied a threshold based on minimum length criteria (unit size/minimum repeat time): six for mononucleotide and dinucleotide, five for trinucleotide, and four for tetranucleotide, pentanucleotide, and hexanucleotide repeats, respectively. Based on the flanking regions of the repeat regions, primers for 68 cpSSR loci were designed using Primer v.7.0 software [16]. The parameters for designing PCR primers were as follows: (1) primer length (18 to 22 bp); (2) PCR product size (100-300 bp); (3) melting temperature between 50°C and 70°C, with 55°C as the optimum annealing temperature; and (4) GC content (40-60%), with an optimum of 50%. After primer design, amplification efficiency and polymorphism were evaluated using seven B. hainesii samples.
2.3. DNA extraction
Leaf samples were rinsed with deionized water and ethanol (70%), and the total genomic DNA was extracted from the plant DNA Kit according to the manufacturer's instructions (BioTeke, Beijing, China). The DNA purity and integrity were tested by Nanodrop ND-2000 spectrophotometer (NanoDrop Technologies, DE, USA) and then diluted to 20 ng‧µl-1.
2.4. cpSSR analysis
The SSR-PCR was performed in a 25 µl reaction volume, comprising 2.5 µl of template DNA, 12.5 µl of 2X Taq Master mix, 1 µl of each primer, and 8 µl deionized water. All reactions were performed in a MasterCycler (Eppendorf, Hamburg, Germany) with the following settings: 3 min of initial denaturation at 95°C; 35 cycles of denaturation for 45 s at 95°C, annealing for 45 s at 54°C - 56°C, and extension for 45 s at 72°C; followed by a final extension for 10 min at 72°C. The amplification products were separated using a Sequi-Gen®GT DNA electrophoresis system in 8% (w/v) polyacrylamide gels in TAE buffer and then stained by ethidium bromide for 10 min. The banding patterns were visualized under UV light and photographed using a UV Transilluminator camera (CLEAVER sci. ltd). A 100 bp DNA ladder (Invitrogen) was used as the standard.
2.5. Data analysis
The genetic diversity was estimated based on the SSR allele frequencies, including the number of alleles (Na), number of effective alleles (Ne), Shannon’s information index (I), the observed heterozygosities (Ho), and the expected heterozygosities (He) were calculated with GenAlEx 6.5 [17]. Additionally, the genetic distance matrix between individuals was also computed based on cpSSR data utilizing GenAlEx 6.5. Unweighted Pair Group Method with Arithmetic mean (UPGMA) phylogenetic trees were generated using MEGA 11.0 [18].
3. RESULTS AND DISCUSSION
3.1. Frequency and distribution of cpSSR
We used the recently sequenced chloroplast (cp) genome of B. hainesii [11]. All 164,305 bp were examined to discover microsatellite markers in the cp genome of B. hainesii (Table 1). A total of 133 potential cpSSRs were identified. A total of 1 and 133 sequences had one and more than one microsatellite locus, respectively and the number of cpSSR involved in compound formation (26).
Table 1. Summary cpSSR in the cp genome of B. hainesii
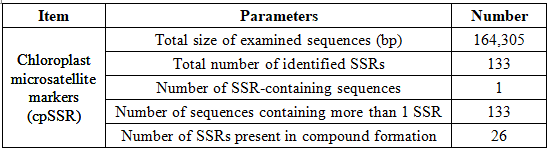
Of the 133 potential cpSSR, distribution to different repeat type classes comprising mononucleotide repeats was the most abundant (121; 91%), followed by dinucleotide repeats (11; 8.3%), and trinucleotide repeats (1; 0.7%) (Table 2). It can be seen that the main repeat types of microsatellite sites in the cp genome of B. hainesii mononucleotide repeats, followed by dinucleotide repeats and trinucleotide repeats.
Table 2. Frequencies of SSR repeat types in the cp genome of B. hainesii
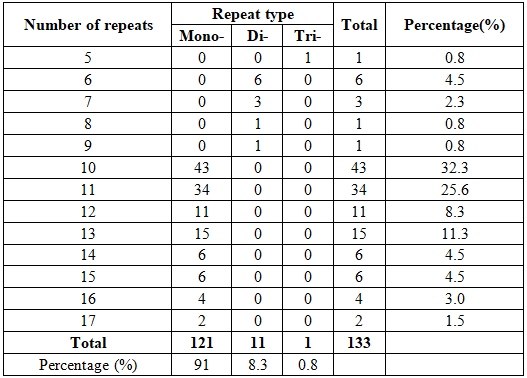
Through statistical analysis of cpSSR based on motif types in the cp genome of B. hainesii, the results are shown in Table 3. In the mononucleotide repeats, A/T had a large proportion (88% of all SSRs), followed by C/G (3%). In the dinucleotide, the dominant nucleotide repeats were AT/AT (7.5%), followed by AG/CT (0.8%). Only 1 (0.8%) trinucleotide AAT/ATT was observed in the species.
Table 3. Frequencies of cpSSR motif types in the cp genome of B. hainesii
Microsatellite motif | Number of repeats | Total | Percentage (%) | ||||||
5 | 6 | 7 | 8 | 9 | 10 | > 10 | |||
A/T | - | - | - | - | - | 42 | 75 | 117 | 88 |
C/G | - | - | - | - | - | 1 | 3 | 4 | 3.0 |
AG/CT | - | 1 | 0 | 1 | 0.8 | ||||
AT/AT | - | 5 | 3 | 1 | 1 | 0 | 10 | 7.5 | |
AAT/ATT | 1 | 1 | 0.8 |
3.2. Genetic diversity
The 68 pairs of cpSSR primers were designed by Primer 7.0 based on 133 cpSSR (Table 4). Of the 20 pairs randomly tested 9 pairs produced PCR products of the expected size and revealed allelic polymorphism, which were used to investigate the genetic diversity of B. hainesii (Table 5, Fig. 2). In the present study, a total of 21 alleles were detected across nine cpSSR loci from 7 adult trees of B. hainesii. The number of observed alleles (Na) ranged from 2 to 3, effective number of alleles (Ne) ranged from 1.2 to 2.0, Shannon’s information index ranged from 0.14 and 0.71. The mean values of the observed (Ho) and expected heterozygosity (He) were 0.25 and 0.27, respectively (Table 5). This result showed the natural populations of B. hainesii maintain a low genetic diversity level. The results of this study are similar to previously reported low genetic diversity among populations of R. apiculata (Rhizophoraceae) (He = 0.352) using microsatellite markers [6]. B. gymnorrhiza (H = 0.027-0.475) and Kandelia candel (H = 0.175-0.370) by cpSSR [14]. Genetic diversity is important because it gives species a better chance of survival. However, genetic diversity can be lost when populations get smaller and isolated, which decreases a species' ability to adapt and survive. If genetic diversity gets too low, species can go extinct and be lost forever. This is due to the combined effects of inbreeding depression and failure to adapt to change. In such cases, the introduction of new alleles can save a population. During field surveys in 2023 and 2024, we found the population size of B. hainesii very small with only 7 individuals with more than 20 cm diameter (dbh). UPGMA tree for 7 B. hainesii accessions was constructed based on genetic distance data showed that all individuals had a close genetic relationship and were divided into two groups (Figure 3). The first group included three individuals (VH1, VH4, and VH7) and the second group included four individuals (VH2, VH3, VH5, and VH6). Notably, B. hainesii is considered a hybrid between B. gymnorhiza and B. cylindrica [12, 13, 19] rare hybrid individuals can reproduce successfully. Thus, these trees should be protected as an important genetic resource bank which makes it necessary to continue with asexual reproduction experiments (propagation of B. hainesii by Air-layering, grafting, stem cuttings, micropropagation).
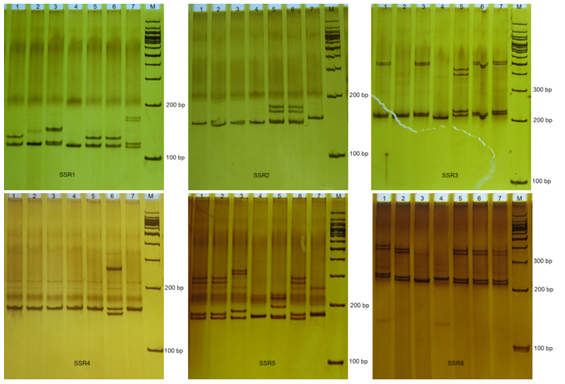
Figure 2. Gel pictures of seven B. hainesii genotypes produced with some primer pairs SSR (lane M is a 100bp ladder and lanes 1 to 7 represent different)
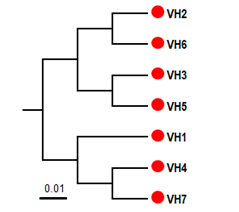
Figure 3. UPGMA phylogenetic tree of 7 individuals B. hainesii
Table 4. List of primers pairs designed for B. hainesii
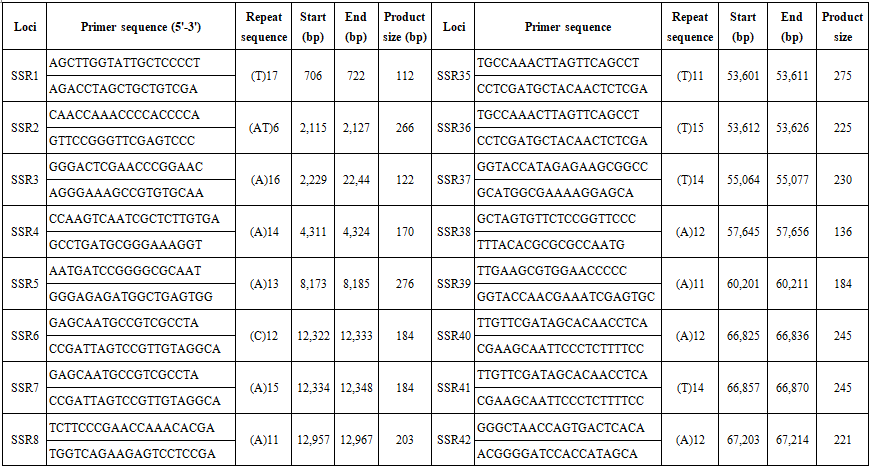
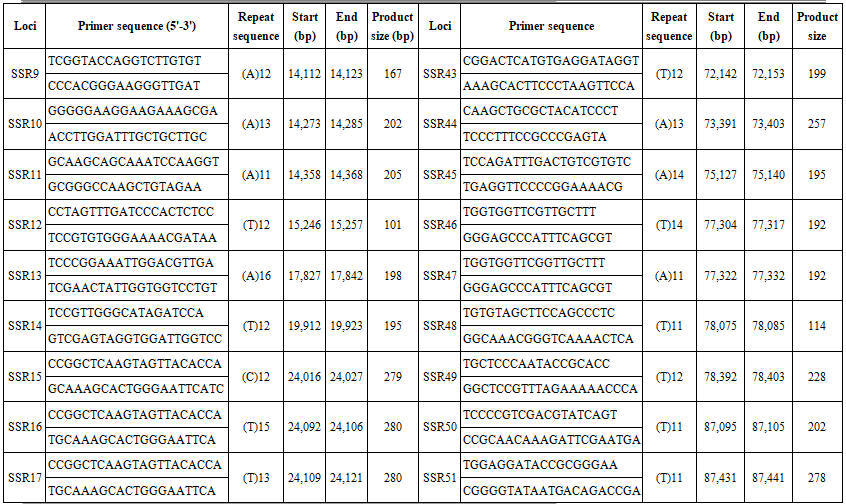
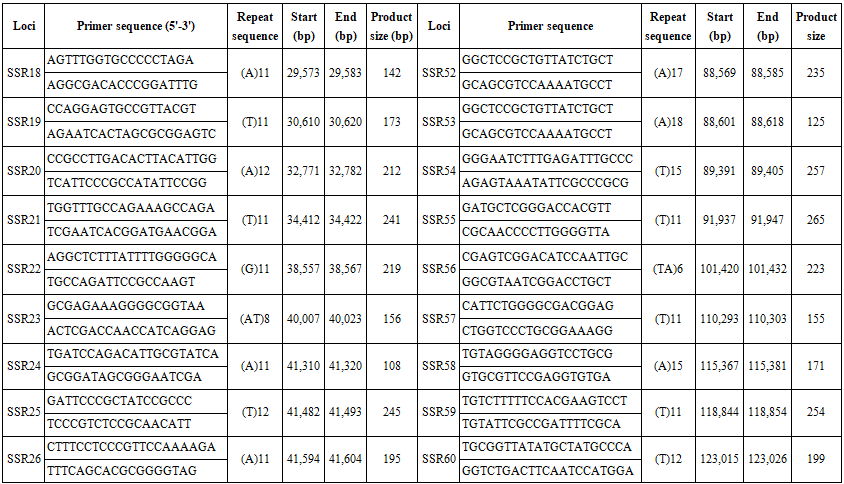
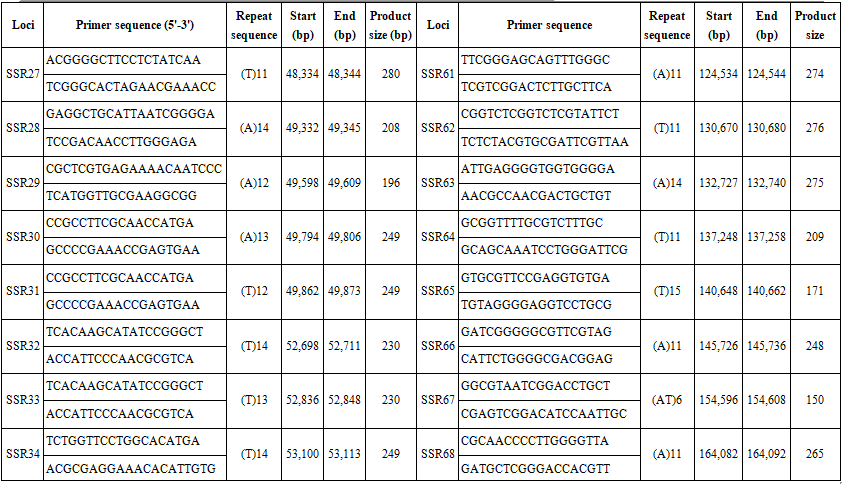
Table 5. The characteristics of nine polymorphic chloroplast microsatellite loci developed for B. hainesii
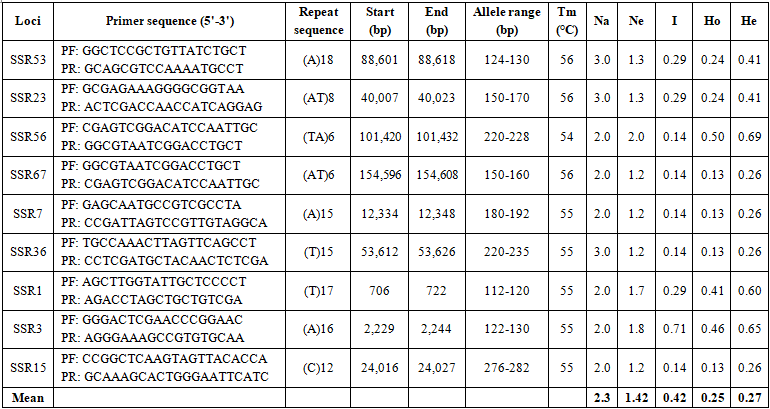
Note: Na = No. of different alleles; Ne = No. of effective alleles; Ho = observed heterozygosity; He = expected heterozygosity; I = Shannon’s information index.
4. CONCLUSIONS
We developed and characterised nine chloroplast microsatellite markers using the recently sequenced cp genome of B. hainesii. These cpSSR markers were subsequently employed to identify low genetic diversity of B. hainesii. The polymorphic cpSSR primers are expected to be beneficial in intra- or interspecific genetic studies of the genus Bruguiera.
Acknowledgments: This research was funded by basis project of Joint Vietnam-Russia Tropical Science and Technology Research Center (2023-2025).We are grateful to the directorates of the Con Dao NP for their support of our fieldwork and for issuing relevant permits.
References
2. Vien Ngoc Nam, Tran Dinh Hue, Mangrove Flora Biodiversity in Con Dao National Park, Ba Ria - Vung Tau Province, Vietnam, Proceedings of International Conference on Coastal Environment and Management, Nagoya University, Japan, 2009, p.96-104.
3. Duke N., Kathiresan K., Salmo III S. G., Fernando E.S., Peras J.R., Sukardjo S., Miyagi T., Ellison J., Koedam N.E., Wang Y., Primavera J., Jin Eong O., Wan-Hong Yong J., Ngoc Nam V., Bruguiera hainessii, The IUCN Red List of Threatened Species, 2010.
4. Cooper W. E., Kudo H., Duke N. C., Bruguiera hainessii C.G. Rogers (Rhizophoraceae), an endangered species recently discovered in Australia, Austrobaileya, 2016, 9(4):481-488. http://www.jstor.org/stable/44648648
5. Yan L., Fan P., Wambulwa M., Qi H., Chen Y., Wu Z., Milne R., Khan R., Luo Y., Gao L., Shen S., Rashid I., Khan S. M., Maity D., Li D., Liu J., Human‐associated genetic landscape of walnuts in the Himalaya: implications for conservation and utilization, Diversity and Distributions, 2024, 30(4):e13809. DOI:10.1111/ddi.13809
6. Azman A., Ng K. K., Ng C. H., Lee C. T., Tnah L. H., Zakaria N. F., Mahruji S., Perdan K., Abdul-Kadir M. Z., Cheng A., Lee S. L., Low genetic diversity indicating the threatened status of Rhizophora apiculata (Rhizophoraceae) in Malaysia: declined evolution meets habitat destruction, Sci. Rep., 2020, 10(1):19112. DOI:10.1038/s41598-020-76092-4
7. Abeysinghe P. D., Triest L., De Greef B., Koedam N., Hettiarachi S., Genetic differentiation between Bruguiera gymnorhiza and B. sexangula in Sri Lanka, Hydrobiologia, 1999, 413:11-16. DOI:10.1023/A:1003899028558
8. Basyuni M., Baba S., Oku H., Microsatellite analysis of genetic diversity and structure of Bruguiera gymnorrhiza and Kandelia obovate, E3S Web of Conferences, 2018, 52:1-10. DOI:10.1051/e3sconf/20185200027
9. Ebert D., Peakall R., Chloroplast simple sequence repeats (cpSSRs): technical resources and recommendations for expanding cpSSR discovery and applications to a wide array of plant species, Molecular Ecology Resources, 2009, 9(3):673-690. DOI:10.1111/j.1755-0998.2008.02319.x
10. Shi C., Han K., Li L., Seim I., Lee S. M. Y., Xu X., Yang H., Fan G., Liu X., Complete chloroplast genomes of 14 mangroves: phylogenetic and genomic comparative analyses, BioMed Research International, 2020, p.1-13. DOI:10.1155/2020/8731857
11. Tran V. H., Hoang T. T. T., Pham M. P., Vu D. G., Nguyen Q. K., Vu D. D., Complete chloroplast genome of endangered Bruguiera hainesii C.G Rogers 1919 and phylogenetic analysis with associated species, Biomedical and Biotechnology Research Journal, 2023, 7(4):590-597. DOI:10.4103/bbrj.bbrj_218_23
12. Ruang-Areerate P., Kongkachana W., Naktang C., Sonthirod C., Narong N., Jomchai N., Maprasop P., Maknual C., Phormsin N., Shearman J. R., Pootakham W., Tangphatsornruang S., Complete chloroplast genome sequences of five Bruguiera species (Rhizophoraceae): Comparative analysis and phylogenetic relationships. Peer. J., 2021, 9:1-22. DOI:10.7717/peerj.12268
13. Shearman J. R., Naktang C., Sontirod C., Kongkachana W., U-thoomporn S., Jomchai N., Maknual C., Yamprasai S., Promchoo W., Ruang-Areerate P., Pootakham W., Tangphatsornruang S., Assembly of a hybrid mangrove, Bruguiera hainesii, and its two ancestral contributors, Bruguiera cylindrica and Bruguiera gymnorhiza, Genomics, 2022, 114(3):110382. DOI:10.1016/j.ygeno.2022.110382
14. Islam M.S., Lian C., Geng Q., Kameyama N., Hogetsu T., Chloroplast microsatellite markers for the mangrove tree species Bruguiera gymnorrhiza, Kandelia candel, and Rhizophora stylosa, and cross-amplification in other mangrove species, Conservation Genetics, 2007, 9(4):989-993. DOI:10.1007/s10592-007-9424-x
15. Thiel T., Michalek W., Varshney R. K., Graner A., Exploiting EST databases for the development and characterization of gene-derived SSR-markers in barley (Hordeum vulgare L.), Theor. Appl. Genet., 2003, 106(3):411-22. DOI:10.1007/s00122-002-1031-0
16. Clarke K.R., Gorley R.N., PRIMER v7: User Manual/Tutorial. PRIMER-EPlymouth. 296p, 2015.
17. Peakall R., Smouse P. E., GenAlEx 6.5: genetic analysis in excel. Population genetic software for teaching and research an update, Bioinform., 2012, 28:2537-2539. DOI:10.1093/bioinformatics/bts460
18. Tamura K., Stecher G., Kumar S.. MEGA11: Molecular evolutionary genetics analysis version 11, Mol. Biol. Evol., 2021, 38(7):3022-3027.
19. Ono J., Yong J. W., Takayama K., Saleh M. N. B., Wee A. K., Asakawa T., Yllano O. B., Salmo S. G., Suleiman M., Tung N.X., Soe K. K., Bruguiera hainesii, a critically endangered mangrove species, is a hybrid between B. cylindrica and B. gymnorhiza (Rhizophoraceae), Conservation Genetics, 2016, 17(5):1137-1144. DOI:10.1007/s10592-016-0849-y